Research Profile: Professor Michelle Coote
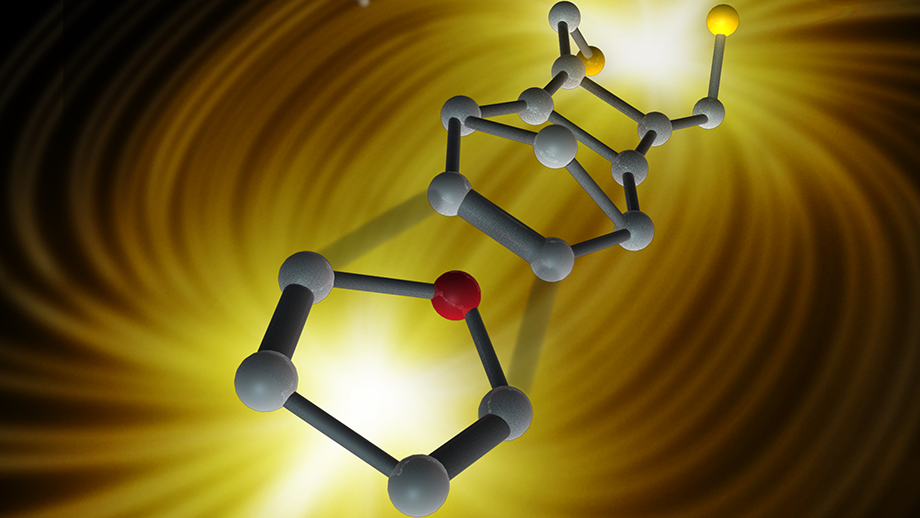
Understanding how and why chemical reactions occur has always been of huge importance. In particular, chemical reactions are essential to the development of many of the materials that surround us such as plastics and functional materials.
While we have great knowledge of these processes we still have a long way to go. One researcher at the Research School of Chemistry — Professor Michelle Coote — alongside her research team, are making leaps and bounds in our understanding of chemical reactions.
“I’ve always been interested in why chemical reactions happen the way they do and how we can change the outcome,” Michelle says. “The main interest of our group is designing new reagents and catalysts to control chemical reactions. We do this using a combination of theory and experiment.”
Michelle’s team is taking advantage of computational quantum chemistry to create a better understanding of chemical reactions. High speed computers located within ANU allow the team to develop predictions about the chemical nature and structure of particular molecules, and in turn develop theories as to why reactions occur in the way they do. From here her team can take these predictions to the lab to see if they have gotten it right.
Using this process Michelle’s team is fundamentally changing some of our understandings of the chemical process. In particular, they are examining the role of electric fields in chemical reactions, upending many of the assumptions of modern chemistry.
“The project we’re most excited about is one to use electric fields to control chemical reactions,” Michelle explains.
“In chemistry there is something known as a ‘redox process’, which is a process that involves an actual exchange of electrons. It’s well known that electric fields change that process. However, among experimentalists it is not well accepted that a non-redox process can also be manipulated by an electric field.
It is this understanding that Michelle’s team wants to upend.
“From doing theory we know that in any process, even if it’s not a redox process, most molecules will have some degree of polarity in them. One end will be delta minus and one end will be delta plus. So theoretically it makes sense that an electric field, if it’s oriented the right way, would stabilise one molecule or destabilise another. Electric fields should be able to influence chemical reactions by having disproportionate effects on one species over another.”
Through a mixture of theory and experiment, Michelle and her team are starting to prove this theory. Her team are using two separate research processes to do so. The first is a process called “pH switching”, in which the team uses molecules that have either acids or bases on them. These molecules will either have a positive or negative charge, with the charge having an electric field associated with it. Within these molecules Michelle and her team have managed to switch this charge, proving the role electric fields can play in chemical reactions.
The second technique is to actually use an electric field, which as Michelle says is “kind of cooler”. For this process Michelle and her team are working with collaborators from the universities of Wollongong and Barcelona who use a technique called ‘scanning tunneling microscopy’. The teams use a gold surface and gold tip, which are very close to each other, and tags molecules to the surface and tip, in doing so the team can then measure the conductivity between the surface and tip, which can be used to detect chemical reactions. Through managing the strength of the electric field within this process, the team can then measure the impact the electric field has on the chemical reaction. What the team has found is that the electric field increased the reaction rate in one direction, and had no effect on the reaction rate in the other. This is exactly what Michelle and her team predicted.
Both the theoretical predictions and experimental verification have led to a fundamental change in our understanding of how chemicals reactions occur. While theoreticians have believed that electric fields could impact chemical reactions, Michelle and her team are the first to prove it. This will have major impacts.
As Michelle says:
“Some theoreticians have known this for some time, but we are certainly the first to prove it. It makes you start seeing these electrostatic affects everywhere. A lot of catalytic effects that people have been using already probably has an electrostatic component. Once you recognise that you can enhance the electrostatics you can enhance the catalysis, so it’s kind of a new tool for catalysis design.
“For example, in regards to the pH switching, what we’re trying to do is create a reaction that could not take place at room temperature without an electric field. But with an electric field, due to our pH switch, we can turn the reaction on at room temperature and then turn it off again. This will mean materials companies can work at lower temperatures, which means they get fewer side reactions. They also have a much nicer way of controlling it.”
This control could result in significant changes in material production, making the entire process much more streamlined and easier to manage. The results of this fundamental research will have a long term impact in research and industry.