Revealing the power of nature's sparkplug
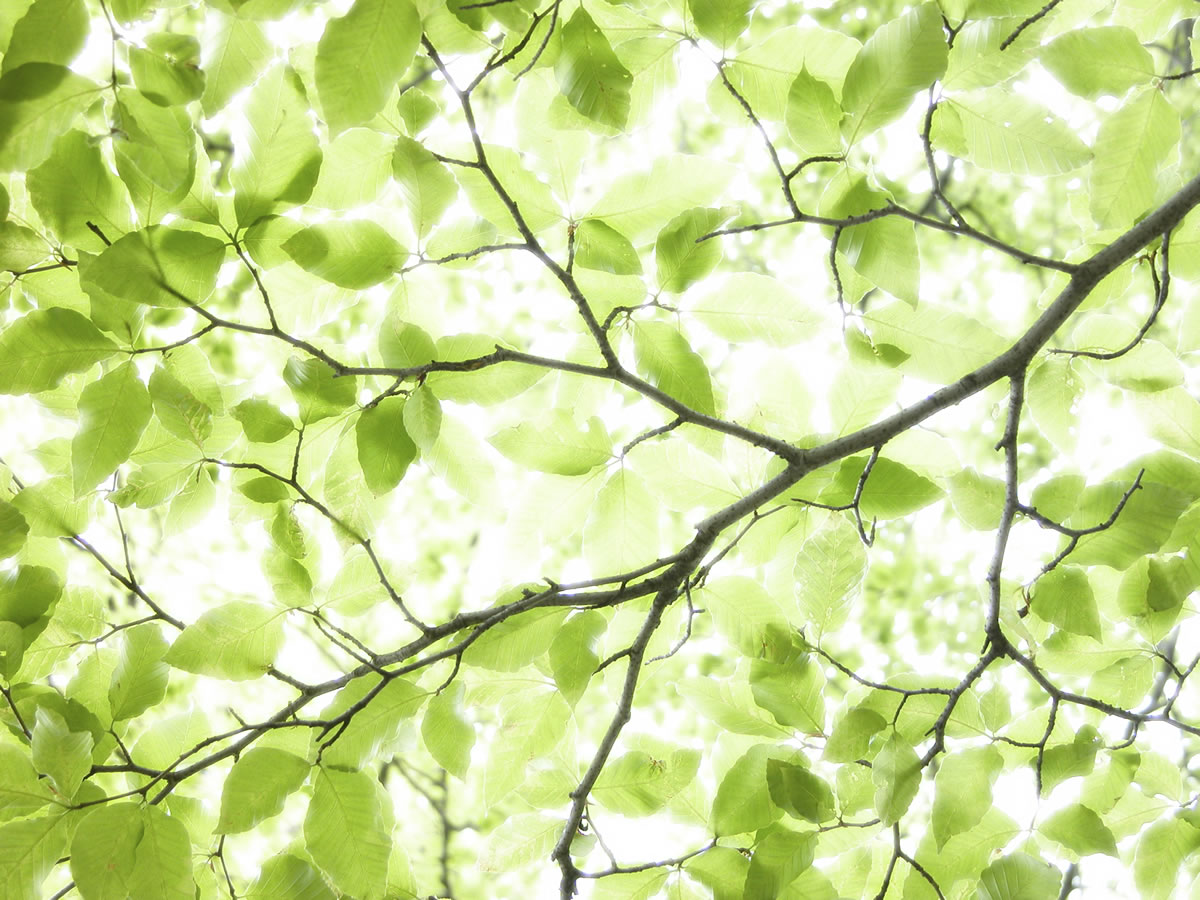
Scientists have used computer modelling to reveal the complex chemistry behind one of nature’s best kept secrets—the chemical sparkplug that plants use to make energy.
New research from the Australian National University (ANU) shows that the chemical reaction site in plants, where sunlight is used to convert water into oxygen and hydrogen, requires much less chemical pulling power than previously thought.
These results not only change our understanding of how plants make energy, but they could also help guide future technologies aiming to make clean, renewable hydrogen fuel for use in planes and cars.
As Professors Rob Stranger and Ron Pace explain, in chemical terms this pulling power, corresponds to the manganese oxidation state needed to strip electrons from water. Understanding this is an important foundation to mimicking this process to make hydrogen.
“Over billions of years, this chemical reaction has operated at almost maximum efficiency,” said Professor Rob Stranger from the Research School of Chemistry.
“By proving that this reaction occurs at a lower oxidation state than currently accepted, we have shown just how effective nature can be at operating at the outer limits of chemical possibility.”
When this complex chemistry evolved 3 billion years ago it changed the Earth’s atmosphere forever. But despite producing enough oxygen for both us and the dinosaurs to breathe, it is one of the most dangerous reactions in nature. If the chemical sparkplug has too much pulling power it could cause a lot of damage.
The problem is like “setting fire to a wicker basket without burning the basket” explains Professor Ron Pace also from the Research School of Chemistry.
This new evidence further dispels the traditional scientific view that the reaction must require more pulling power involving manganese atoms in a higher oxidation state. And the ANU researchers believe a paradigm shift towards the low oxidation state model is needed before we can mimic this photosynthetic process to produce hydrogen fuel.
“Over the past 20 years there has been a vigorous debate about the nature, structure and mechanism of this key catalytic site called the water-oxidizing-complex,” said Professor Pace.
“The matter come to a head in 2011 when high-resolution X-ray crystal structures of the system emerged which pointed to a lower manganese oxidation state which confused everyone—but not us.
“This posed such a threat to the traditional view that some people suggested that the crystals must have been perturbed from the X-ray exposure making it appear that the oxidation state of the manganese atoms was lower.”
But early this year, the same group in Japan produced a new crystal structure by reducing the X-ray exposure with similar results.
The researchers from the ANU then used the super-computer at the National Computational Infrastructure facility, to model the chemical structure of both crystals.
“Remarkably, we found that both crystal structures are just two closely related members of the same family, and they both have the same low manganese oxidation state. In fact the only difference was the location of a single proton,” said Professor Stranger.
“This is very significant because we have now have the keys needed to finding out exactly how the water is oxidised in plants at the molecular level. Once we know how the mechanism works the next step will be to mimic it in the most efficient way possible.”
This research is published in Angewandte Chemie.